O-ring seals are the standard in virtually all industries and applications. Fitted in seal grooves, they allow for easy installation and replacement when they show signs of wear. What is an undeniable advantage for static seals (which seal non-moving parts) quickly finds its limits with dynamic seals (which seal moving parts). This is especially true when it comes to demanding applications with high requirements on sealing performance as well as long-term stability.
Many applications of vacuum valves have the same requirements: excellent sealing properties even at high differential pressures, good damping behavior to avoid shocks, especially during repeated, fast closing movements, and high long-term stability to minimize downtime due to maintenance.
O-rings in such dynamic sealing applications face some challenges here. For example, when very large O-rings are used, they tend to twist, which limits their sealing ability. In addition, O-ring grooves are associated with the risk of enclosed gas volumes, i.e. areas under the O-ring that are sealed off from the rest of the process and thus from pressure changes. These volumes pose a risk. If, for example, the ambient pressure drops due to the process, the pressure difference to the pressure in these areas increases. The differential pressure can then become so great that the O-ring is forced out of the groove, which negatively alters the sealing properties, up to a total loss of the sealing capacity. Vacuum valves with dynamic O-ring seals therefore usually have special O-ring grooves to counteract this.
Bonded seals do not have these problems because they are fixed directly to the surface of a closing plate. This direct attachment has the added advantage that all of the elastomer material used for the seal is included in the sealing process, whereas with an O-ring solution, some of the material must be used to hold the seal in the groove.
Since elastomers are generally a source of particles and outgassing in vacuum processes, less material used means fewer particles and less outgassing. Bonded seals use on average 35% less material compared to similar O-ring solutions.
Because direct bonding uses all of the material for the sealing function, there is also a higher degree of influence on the design of the sealing properties. This means that bonded seals can be tailored from the first contact with the seal seat to the final position on the sealing surface. By changing the height and width of the seal, for example from a pointed to a flat pyramid shape, or by rounding the tip at different radii, the damping effect of the seal when the valve closes can be controlled. The design can also influence the opening behavior. For example, in the case of large differential pressures, the design of the bonded seal in addition to the valve control can dampen an opening pressure pulse or avoid it altogether. This is primarily relevant for vacuum control valves or generally for valves with mechatronic actuators. Here, appropriately designed bonded seals can be used to permit small, metered volume flows with a controlled slight reduction in closing pressure, which dampens a change in pressure. This increases the control quality and the controllable conductance spectrum of the valves.
Bonded seals, like O-ring seals, use different elastomers with different properties to withstand different process conditions such as aggressive chemicals, high or low temperatures, or other demanding conditions.
For bonded seals, this creates the challenge of bonding these materials to the plate surface with the same bond quality every time. However, since some elastomer materials differ greatly in their bonding properties, not all can be bonded using the same technology. VAT therefore uses various chemical and physical bonding methods.
In chemical bonding, a bonding agent is applied between the sealing material and the metal surface of the plate. The challenge is to find a binder that has the same chemical and physical resistance and aging performance as the seal material itself. Furthermore, the bonding performance must not fall below the sealing performance of the seal material; otherwise, the bond would become the weak point and determine the service life of the seal, instead of the seal material itself.
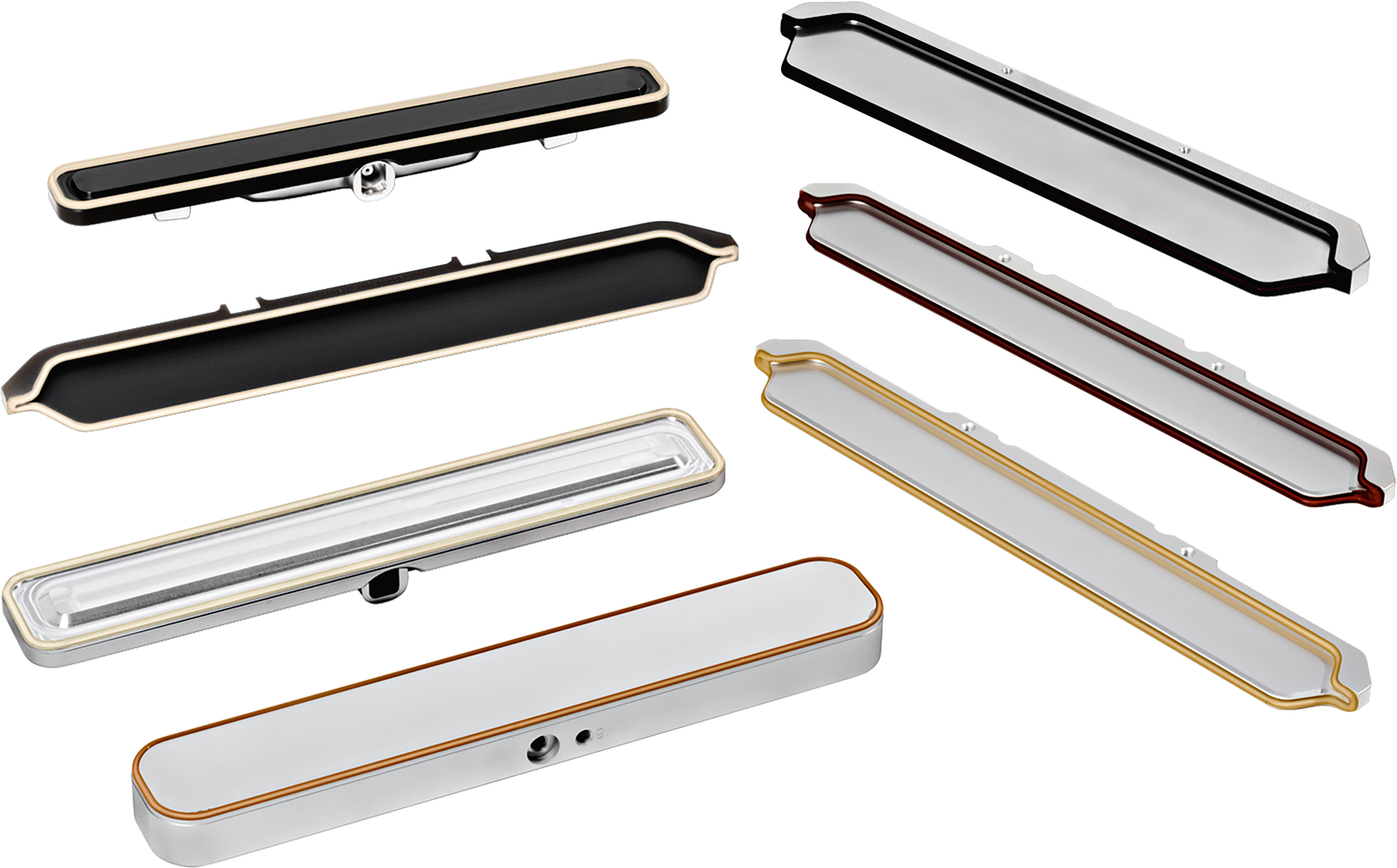
To ensure this does not happen, VAT uses an extensive database of seal materials, binders and combinations of the two under various process conditions, built up over many years. The goal of this database, in addition to its primary function of providing optimal combinations for specific conditions, is to identify areas for improvement and the development of new bonding solutions.
A growing alternative to chemical bonding is physical bonding. Simply put, physical bonding works like Velcro, where one side hooks into the other side or both sides hook into each other. The challenge is that, unlike Velcro, different materials with significantly different properties in terms of elasticity and fracture behavior meet on each side. Developing a reliable hook-and-loop-like connection therefore requires some special knowledge. For example, a targeted optimal interlock is designed to provide a permanent rather than a removable connection, and ensures that this connection is durable enough to maintain constant sealing properties over its lifetime, even when subjected to high dynamic stresses and differential pressures.
VAT has its own development team dedicated to researching and developing this bonding technology, the main advantage of which is that, unlike chemical bonding, no third material is required.
However, the focus is not only on improved bonding between the seal material and the surface of the valve plate. The team is also looking at the other side, the seal seat. The sealing performance of bonded seals, as well as O-ring seals, is determined not only by the shape and selection of the seal materials and their attachment to the closing plate, but also by the interaction with the seal seat or, more precisely, with the surface and material of the seal seat. Therefore, the surface design on the vacuum valve seat is another integral part of the design of high-performance seals and, in particular, high-performance bonded seals.
The challenge here is to find the right balance between seal performance and avoidance of bonding behavior or sticking. In a very effective seal, the elastomer material almost completely fills all the micro-depressions provided by the surface of the seal seat. However, the better the elastomer material bonds with the surface roughness of the valve seat, the better it seals, and the more it tends to stick to the surface, which in turn limits sealing performance. To avoid this, a special surface finish is used to greatly reduce the roughness and thus the number of micro-depressions. However, this is not a complete solution, since every elastomer material itself has a certain "roughness," meaning it has small depressions and elevations in its surface. When the rough elastomer surface meets a smooth seating surface, the adhesion behavior decreases, but the existing "gaps" are not completely closed, even if the sealing surface is increased (e.g., by increased pressure on the seal or by design). The challenge, then, is to design the sides to maximize positive engagement without causing adhesion, and to do so without increasing the amount of material used and without dynamically overpressing the seal, both of which have negative effects: reduced seal life and increased risk of particle emissions.
An important dimension in the design of bonded seals is also temperature. In principle, the elastomer materials used are always optimized for specific temperature ranges. Nevertheless, the way in which temperature changes affect the seal is crucial (i.e., through slow changes or short temperature peaks). The "temperature profile" of a process (specifically, the effect of temperature changes on the seal over time and in relation to the dynamic load on the seal) is quite critical to seal performance and life. Temperature changes elastomer behavior even in the approved temperature range. To put it simply, seals become softer or harder. What matters is not only that they do, but also when. Dynamic sealing performance is most required during the closing process and during the sealing process, and this should always happen in the optimum temperature range. This also guarantees optimum particle prevention performance.
Therefore, when selecting a seal, in addition to the chemical conditions and the maximum and minimum temperatures of the process, attention must be paid to which temperature profile is encountered. If the profile is known, the use of passive or active cooling or heating measures can, if necessary, sustainably improve seal performance.
VAT has already successfully met this challenge in the design of bonded seals for many applications, motivating a large number of customers to switch to bonded seals. This is especially true when it comes to high demand for durability and stable, reliable sealing behavior. The VAT development team continues to see great potential for further adapting the technology used to different process conditions and applications.