Since its discovery by Otto Hahn and Lise Meitner in 1938, nuclear energy has had an extremely eventful history. Hiroshima and Nagasaki became symbols of the immense scale of destruction that the fission of atomic nuclei can unleash. But the period immediately after World War II is also emblematic of the enormous enthusiasm that the possibility of using nuclear energy peacefully to meet the world's energy needs sparked. No stinking combustion fumes, virtually unlimited amounts of energy, reliable electricity at any time of day - all of this sounded too good to be true to the people of the time.
From the very beginning, researchers warned that some types of reactors, such as uranium-based light-water reactors, would produce highly radioactive residues with enormously long half-lives, and that their safe storage or sensible further use (e.g. for military purposes) would have to be urgently considered. However, for a variety of reasons, including political ones, innovative alternative reactor technologies have not been able to establish themselves on a large scale. Uranium-based light-water technology has long played a leading role in the peaceful use of nuclear power and the result of this is the fact that long-radiating decay products is a problem.
Only 4 percent of the energy originally contained in the nuclear fuel is used in this type of energy generation, and as such, the nuclear "waste" that is generated still contains an incredible 96 percent of usable nuclear energy. About 12,000 tons of this nuclear waste are produced in reactors worldwide every year. The recent abandonment by many countries of breeder technology, originally hailed as a ray of hope because in breeder reactors, some of the decay products are also fissionable and thus reusable for renewed energy production, makes the question of the final storage of nuclear waste all the more urgent. The problem is the fact that a suitable storage site would have to be stable for about one million years to ensure that no fission product would radiate dangerously - that's longer than the entire history of mankind to date!
No question, we will require storage sites for nuclear waste. But as with conventional landfills, what you choose to store there makes a huge difference. For example, a composting facility for your organic waste will certainly require far fewer safety precautions than, say, a hazardous waste site for leftover paint or other chemicals. Accordingly, researchers are working feverishly on new technologies to "defuse" or even completely recycle highly radioactive nuclear waste.
One particularly promising process for this is transmutation. Whereas the term originated in medieval alchemy and described the transformation of base metals such as mercury into precious metals such as gold, in the modern context transmutation is understood to mean a process in which spent reactor fuel rods are bombarded with very energetic neutrons, as a result of which nuclides with particularly long half-lives (such as plutonium, neptunium or curium) split into isotopes with a significantly shorter lifetime. It is true that these so-called transuranium isotopes make up only a comparatively small proportion of the mass of nuclear waste. However, the key is that these nuclides are the most problematic because they emit a particularly large amount of radioactive radiation for a particularly long time. If we were to find a sustainable recycling solution for these substances, we would be a huge step ahead in the question of nuclear waste disposal. It is estimated that the half-life of nuclear waste could be reduced to less than 1,000 years by transmutation - still numerous human generations, but much more manageable than the millions of years just mentioned!
Over the years, two particularly promising transmutation technologies have emerged: accelerator-based systems and reactor-driven systems. They differ primarily in how the fast neutrons needed for transmutation are generated.
In accelerator-driven systems (ADS), the transmutation facility essentially consists of a proton accelerator to generate the fast neutrons and a reactor in which the radiating nuclear waste is then bombarded with the neutrons. You may be wondering now how on earth fast neutrons come out of a proton accelerator? The magic word is spallation. In this process, a beam of protons is first accelerated almost to the speed of light and then directed at a target - for example, a tank containing a liquid lead alloy. The lead nuclei are excited by the hurtling protons to such an extent that they "shake off" twenty to thirty high-energy neutrons per nucleus - and with one sixth of the energy input that you would need to produce the neutrons by nuclear fission.
Speaking of nuclear fission: Another advantage of spallation is that the process is not based on a chain reaction. As soon as you deactivate the proton beam, no more neutrons are emitted. This is one of the reasons that spallation technology is comparatively easy to control. It is no wonder that it has experienced an enormous surge in development in recent years and this has also brought ADS systems into the realm of the technically feasible.
An extensive ADS experimental facility is under construction at the J-PARC accelerator center in Japan, where researchers plan to investigate the fundamental physical properties of transmutation using a proton beam of varying power (10 W to 250 kW). The Belgium-based European research project MYRRHA (Multi-Purpose HYbrid Research Reactor for High-Tech Applications) has also taken up the idea of ADS transmutation. With the aid of a separation process specially developed for this purpose, the long-lived transuranic elements are to be isolated from the spent fuel rods and then broken down into shorter-lived isotopes by transmutation. The first MYRRHA prototype reactor in combination with a 100 MeV proton accelerator for the production of spallation neutrons is scheduled to go into operation in 2026 - ideal conditions to raise transmutation research to new heights.
In reactor-driven systems, on the other hand, the fast neutrons are to a certain extent a by-product of the nuclear fission occurring in the course of atomic energy production. In the breeder reactors already mentioned, one could in principle use the neutrons emitted in the course of nuclear fission directly for transmutation of the remaining transuranics. What is particularly exciting about this approach is that the energy released during transmutation could also be used as useful energy, because the process takes place within the conventional reactor.
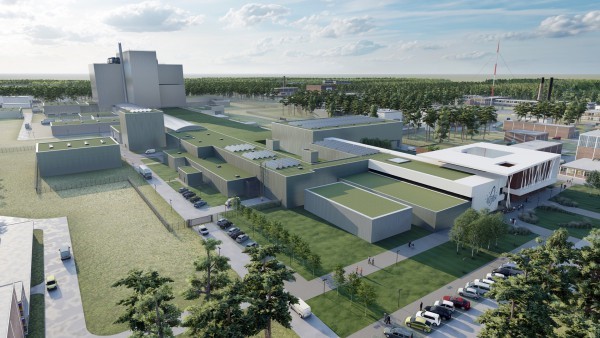
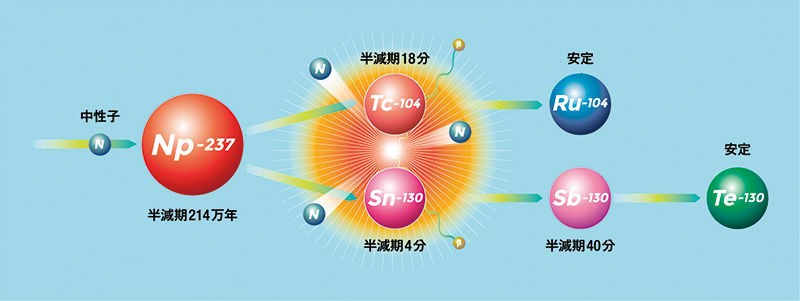
Source: http://j-parc.jp/uploads/2019/genri_800.jpg
There is a good chance that transmutation will reduce the radiotoxicity of nuclear waste and the size and lifetime of repositories by several orders of magnitude. So what are we waiting for? Despite transmutation seemingly being the answer to the problem, it is still in its infancy. So far, only small-scale test facilities have been realized, and there are still many, many, many technical questions unanswered. Also, proof that transmutation technology can be scaled up in an economically viable way has yet to be provided. Experts estimate that it will be at least 20 years before the first transmutation plant begins regular operation - if ever. Nonetheless, the technology is gaining ground and will certainly make a name for itself in the near future.
Due to the highly demanding conditions for transmutation experiments - as is so often the case in high-energy physics - a high vacuum is required. High temperatures and strong radioactive radiation do the rest, demanding everything from the components used. They must be enormously resistant to temperature and radiation, exhibit physically inert behavior, prevent contamination as optimally as possible and much more. VAT's all-metal valves have been setting the standard in this respect for many years.
As an example, the XHV all-metal gate valves of the 48.2 series the are designed for reliable shut-off in UHV and XHV applications with high ambient temperatures of up to 300°C and can withstand radiation of up to 108 Gy. A special feature is the hard-on-hard sealing VATRING technology used. Unlike conventional metal-to-metal sealing systems, VATRING technology allows a very high number of closing cycles (> 100,000). Both the seal and the seal seat are made of stainless steel and are only elastically deformed. Furthermore, the technology allows very strong sealing forces at comparatively low axial forces. This minimizes wear and permanently increases the reliability of the hermetic seal. With customized solutions like these, VAT supports various transmutation research projects worldwide.
"We at VAT are highly motivated to use our entire vacuum know-how to make transmutation a real success story," says Evert van der Wiel, VAT Sales Manager, who is looking optimistically to the future.