In December 2022, scientists at the National Ignition Facility (NIF) at Lawrence Livermore National Laboratory in California caused a media sensation when it was announced that in their fusion experiments, they had apparently produced for the first time a so-called burning plasma, i.e., a state in which the energy put into the plasma is compensated by the heat of the helium nuclei produced during fusion. Specifically, they released 3.15 megajoules of fusion energy from a pellet filled with the hydrogen isotopes deuterium (D) and tritium (T), while the laser's energy consumption was only 2.05 megajoules – unquestionably a huge step for the future of nuclear fusion energy production! Earlier in 2022, researchers at the JET Laboratory in the United Kingdom had already made news when they broke their own world record for the amount of energy generated by nuclear fusion: about 59 megajoules of energy for five seconds. These two research results alone speak a clear language: nuclear fusion research is developing rapidly, and nuclear fusion holds huge potential as a safe and sustainable energy source of the future!
Various technological approaches to get the nuclear fusion started have turned out to be promising candidates for the fusion reactors of the future. Thus, the dream of a fusion reactor that continuously generates more energy than it consumes is moving closer, step by step. Of course, there is still a very long way to go: optimistic estimates suggest that the first commercial reactors will not be available until around mid-century at the earliest. Nevertheless, the field has broadened, and more and more players are entering the nuclear fusion playground. For example, in addition to large-scale research projects such as ITER, JET or NIF, more than 30 privately financed companies around the world have now specialized in fusion concepts with commercial potential. Whether research institutes or young start-ups, many of them use valve technology from VAT to build their fusion devices, from customized one-offs to complete valve portfolios.
The so-called Lawson criterion is decisive for the success of a nuclear fusion reaction. Only if this mathematical-physical condition is fulfilled, the fusion reaction is self-sustaining in a macroscopic amount of fuel, because the fraction of the released fusion power remaining in the plasma is larger than the power loss of the plasma. In this case, physicists refer to plasma ignition. If the aforementioned NIF research results prove to be robust, this would be the first time in the history of fusion research that the Lawson criterion has been met in a controlled reaction – a real milestone!
Nuclear fusion is an extreme undertaking in every respect. For two atomic nuclei to be able to fuse with each other, they must first have a very, very high kinetic energy, otherwise they will be kept apart by the repulsive Coulomb forces and do not get into the position to "enjoy" the short-range nuclear force, which is ultimately responsible for fusion and the associated (binding) energy gain. Accordingly, extremely high temperatures and pressures are necessary for fusion experiments. Under these conditions necessary for fusion, hydrogen exists as a plasma, i.e., the electrons have separated from the atomic nuclei and the elementary particles move independently of each other. This plasma must be kept "together" in nuclear fusion reactors and then heated in a controlled manner until the fusion of the atomic nuclei begins. Direct confinement in vessels is out of the question because the plasma would cool immediately if it came into contact with the wall material. So, what to do? Over the years, scientists have discovered two promising solution strategies.
In magnetic confinement, a few grams of the DT gas mixture are introduced into a toroidal vacuum vessel, many cubic meters in size, and heated to up to 150 million Kelvin, causing the gas to enter the plasma state. The electrons and protons in the plasma are then cleverly held inside a "magnetic cage" with the aid of strong magnetic fields. Fusion research focuses on two different types of facility, namely the tokamak and the stellarator. They differ primarily in how the magnetic field confining the plasma is constructed. While tokamaks produce the magnetic ring fields via an electric current that flows within the plasma, stellarators produce the ring fields solely with the help of external coils. Examples of tokamak reactors include the Joint European Torus (JET) in the UK mentioned earlier in the context of their 2022 world record, the ASDEX upgrade in Garching/Germany, and the planned ITER reactor in France. A promising example of a stellarator is Wendelstein 7-X, operated by the Max Planck Institute for Plasma Physics in Greifswald/Germany. Private companies contributing promising research in this area include Commonwealth Fusion Systems, Tokamak Energy, and Renaissance Fusion. Magnetic confinement technology has a long and correspondingly instructive history of development, going back to the 1940s. Also, the many variations that have already been tested make magnetic confinement a promising technology candidate for future nuclear fusion reactors. Compared to the other technologies, however, the construction/operation of plants with magnetic confinement is extremely expensive, and the question of how to permanently stabilize the plasma is currently still causing researchers quite a headache.
During inertial confinement, a capsule of a few millimeters in size containing the DT mixture is heated extremely rapidly (< 0.1 ns) to a temperature of about 100 million degrees by intense laser radiation. In addition, the fuel is compressed to a pressure > 3∙1015 bar by blasting the capsule shell, so that the necessary fusion conditions are fulfilled. The problem of plasma confinement plays a minor role here, because by the time the expanding plasma thunders against the capsule wall, enough fusion reactions have already occurred. The most prominent example of research facilities based on this fusion principle is the aforementioned NIF. Well-known private companies in this research area include Innoven Energy, First Light and Marvel Fusion. The advantages with inertial confinement are a good heat retention and the comparatively long confinement times. Additionally, a lower energy input for operation and the comparatively simple design of the plants speak in favor of this technology. However, there are still numerous challenges to be overcome around the efficiency, stability and control of the plants.
Magnetized Target Fusion (MTF) or Magneto-Inertial Fusion (MIF) are combinations of the two methods already mentioned. As with the magnetic approach, here the fusion fuel is held together at a fairly low density by magnetic fields, while it is heated to form a plasma. As with inertial confinement, fusion is then initiated by rapidly compressing the target to achieve the necessary density and temperature of the fuel. There is reasonable hope that such a combination will greatly simplify the design of fusion devices. However, the efficiency of MTF plants is lower than expected, and achieving plasma ignition is also proving comparatively difficult. Among the most active companies researching in this area are, e.g., Helion Energies and TAE in the USA or Generalfusion in Canada.
Regardless of the specific technology used, nuclear fusion opens up the valuable opportunity of a weather-independent and, above all, emission-free energy source. Another big plus is that hydrogen is a virtually inexhaustible raw material here on earth. In addition, the residues from nuclear fusion are chemically completely harmless, in contrast to the environmentally harmful greenhouse gases produced when fossil fuels are burned. Nor is there any danger of nuclear fusion reactions getting "out of control" like the chain reactions in nuclear power plants: in the worst case, the plasma is incapable of fusion and power generation comes to a standstill. The long-lasting highly radioactive waste so controversially discussed in nuclear power plants also plays a negligible role in nuclear fusion reactors, since mainly non-radioactive helium gas is purged. Although radioactive substances are used in the form of deuterium and tritium, and high-energy neutrons are also involved in the fusion process, they are all released in comparatively small quantities, with comparatively short half-lives and a low to medium degree of activation. All in all, the experts agree that the risks involved in nuclear fusion are completely justifiable in view of the tremendous potential of this energy generation technology!
This actually sounds too good to be true, doesn't it? Unfortunately, controlled nuclear fusion for the purpose of energy production is technically extremely challenging, and despite the great progress made in the last year, there is still a long way to go before we can build a fusion demonstrator that can actually produce energy. Most experts believe that nuclear fusion will not be available as a true energy alternative until 2050. There is much, much work to be done before then. Accordingly, it is to be expected that in the next few years, research institutions and universities around the world will join forces to develop nuclear fusion into an energy source that can be really used. Numerous start-ups have also established themselves in the private sector, contributing valuable research around nuclear fusion. For example, the U.S. company Editekk is developing a tungsten-based plasma-facing material (PFM) that should be able to withstand the extreme conditions in a fusion reactor. And the Belgian start-up Magics is developing radiation-resistant integrated circuits and autonomous machines to reliably operate and maintain fusion reactors.
Due to the extremely high temperatures and pressures, as well as the radioactive load, plant engineering in nuclear fusion research is particularly demanding. Because of its extensive experience with countless demanding high-energy physics research projects all over the world, VAT is the ideal partner for this. Whether on the subject of all-metal designs or temperature and radiation shielding for valves, whether customized individual pieces or complete portfolios, for decades VAT has been supplying many of the most influential fusion facilities with the fitting valve technology.
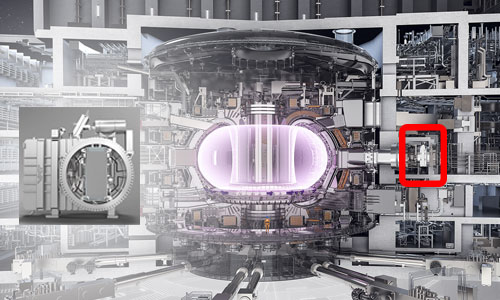
Customized valve solutions in any format: With a diameter of 1.6 meters, the all-metal gate valve manufactured for the ITER project is one of the largest high vacuum all-metal valves ever built. Its main function is to separate the volumes of the tokamak vacuum vessel and the neutral beam vacuum vessel so that both can be vented independently to atmospheric pressure. The technical parameters have it all:
◦ Safety class SIC 1 (earthquake-resistant, among other things).
◦ Weight: 7870 kg
◦ Pressure: 10-9 mbar to 2 bar
◦ Force on the slide plate at differential pressure (2 bar): 27 t
◦ Temperature: up to 200°C
◦ Radiation resistance: 108 Gy
ITER also provides a prime example of the flexibility and solution competence that VAT can fulfill in fusion projects: The all-metal gate valve customized for ITER to separate the tokamak vacuum vessel and the neutral beam vacuum vessel impresses not only with its dimensions, but also with the multitude of technical specifications and tests it had to meet. Phil Schneider, the product manager responsible for all-metal valves at VAT, describes its specific task as follows: "In the ITER reactor, there are three very powerful neutral beam injectors for heating or diagnosing the plasma. Each injector forms a vacuum chamber, which must be capable of being vented independently of the tokamak vacuum vessel for maintenance or in the event of a malfunction." The all-metal gate valve that VAT developed for this purpose is based on innovative VATRING technology - a patented VAT sealing technology for all-metal valves for repeated hermetic closure under UHV conditions. Stainless steel seals with a silver coating are installed in the valve, ensuring high vacuum tightness up to a pressure gradient of 0.2 MPa / 2 bar across the valve disc, with a simultaneous leakage rate of less than 10-7 mbar/s. "For the VAT team, such special developments are an excellent opportunity to contribute their extensive knowledge and many years of experience," says Phil Schneider, pleased with the all-round successful cooperation with the ITER developers.