ELENA – this pretty name stands for Extra Low ENergy Antiproton Deceleration Ring, a 30-m-wide deceleration ring at CERN that slows down the 5.3-MeV antiprotons from the existing CERN antiproton decelerator to a fabulous 0.1 MeV, so that they can, among other things, be captured via so-called Penning magnetic traps and combined with positrons to form antihydrogen.
VAT all-metal vacuum valve technology plays an important role in this, as it maintains full functionality under the operating conditions of high temperature and radiation. The dynamic hard on hard sealing technology combines repeatable hermetic sealing under XHV conditions with an all-metal design.
Examining single antihydrogen atoms
The central goal of ELENA is to keep antimatter alive as long as possible. With great success: Currently, the traps following the ELENA deceleration process achieve antimatter lifetimes between 15 minutes and one year! But also attempts to trap antimatter in so-called cryotraps – i.e., transportable traps with cryostats, where pressures up to 10-18 mbar are reached – are high on ELENA’s agenda (see the PUMA experiment for more details).
In view of the extremely short lifetime of antimatter, the ELENA system must take a wide range of special valve and vacuum technology features into account. For example, all inlets and outlets in the deceleration ring must be completely metal-sealed in order to maintain the required pressures and outgassing values. This is where VAT makes valuable use of its many years of XHV know-how, for example, in the form of the proven all-metal gate valves of the VAT series 48.
Another task that the CERN researchers have on their to-do list: They want to study antihydrogen atoms spectroscopically and then compare the effects of the gravitational force on matter and antimatter. In what is called the GBAR experiment (short for Gravitational Behavior of Antimatter at Rest), the electrically neutral antihydrogen atoms that ELENA helps producing will be dropped from a height of 20 centimeters and then the course of the fall will be recorded until their demise. Thanks to ELENA's excellent ability to keep the antiatoms under investigation as "still" as possible, the GBAR researchers are positive that even the smallest differences in the behavior of matter and antimatter in relation to the comparatively weak gravitational force can thus be detected.
In October 2018, GBAR received its first antiproton beam from ELENA. Since then, the scientists have been working feverishly on research results to get a little closer to solving the great mystery surrounding the asymmetric distribution of matter and antimatter in the universe. In the process, they are also relying on VAT all-metal vacuum valve technology.
The opposite nature of antimatter
But what actually is antimatter? Well, antimatter is matter which consists of antiparticles. And what are antiparticles then? Well, antiparticles are confusingly similar to their matter counterparts, but each has an opposite electrical charge. While protons, for example, are positively charged, antiprotons carry a negative elementary charge. And the positively charged antiparticle to the electron is the antielectron, also called positron because of its positive charge. The existence of such "partner particles" was first postulated in 1928 by the theoretical physicist Paul Dirac. When he examined the Dirac equation – a wave equation based on the special theory of relativity for the description of electrons – in more detail, he was surprised to find that his equation always spat out two possible solutions, one with positive and one with negative energy.
After careful consideration, Dirac concluded that this mathematical curiosity might actually reflect a physical fact by interpreting the two solutions as two different particles: one particle as the familiar electron, and the other particle as an equal-mass antielectron whose opposite charge makes it plausible why the energies of the two particles have different signs.
Antimatter is not a pipe dream!
Sounds bizarre; therefore, it is hardly surprising that Dirac's idea initially met with quite a bit of incomprehension in the scientific community. But only four years later, on August 2nd, 1932, the U.S. physicist Carl David Anderson made a remarkable discovery. At the time, Anderson was working on a detailed analysis of the particle radiation coming from the cosmos. Using a cloud chamber, he visualized the paths of all particles and was thus able to draw valuable conclusions about their properties. In the process, he had noticed a very special particle whose mass corresponded almost exactly to that of the electron, but which had an exactly opposite, positive charge. At first, no one could make sense of this discovery. But soon the experts remembered Dirac and his hypothesis of a positively charged electron. This hypothesis was now confirmed by Anderson's experiment: Anderson had found the antiparticle of the electron!
It soon became clear that every known elementary particle has a “partner”, i.e., an antiparticle. The antiproton was first detected in 1955 during experiments at the Bevatron particle accelerator, and the antineutron followed in 1956, shortly followed by antideuterium, antitritium, and antihelium nuclei. Today, antiparticles are a self-evident part of the so-called Standard Model, which describes all known elementary particles or forces occurring in the universe. So far, all experiments and theories revealed a largely identical physical behavior of matter and antimatter, so they are really "partner particles" and not just coincidental matches of certain parameters.
Matter and antimatter annihilate each other
The fact that particles and antiparticles make "common business" becomes most obvious at the pair annihilation phenomenon. In fact, if an antiparticle meets its material partner particle, the two particles annihilate each other. According to Einstein's famous formula E = mc2, the entire mass of both particles is released as energy – in the case of electron-positron annihilation completely as electromagnetic radiation, and in the case of heavier pairs sometimes also in the form of other particles.
Also vice versa, thanks to Einstein's energy-mass formula, an electron-positron pair can be created quasi out of nothing: from a high-energy, electrically neutral photon, two particles materialize whose mass corresponds to the energy of the underlying radiation and whose opposite charges guarantee that nevertheless the overall electric charge (in this case: no charge) is preserved.
Antiparticles team up as antimolecules
But not only that! Quite analogous to their material partner particles, antiparticles can also join together to form atoms or molecules. Thus, antiatoms have shells of antielectrons and nuclei built of antiprotons and antineutrons.
Light antiparticles such as positrons are observed in natural cosmic radiation, for example. Positrons can also be produced during the decay of radioactive preparations. In fact, this effect is used by physicians to detect tumors at an early stage with so-called PET scanners (short for Positron Emission Tomography). However, since free antimatter reacts with matter within a very short time and is destroyed in the process, heavier antiparticles occur only extremely rarely, and antiatoms or antimolecules do not occur naturally at all. (The heaviest antiatomic nucleus observed so far is antihelium 4, in 2011 during experiments in the Relativistic Heavy Ion Collider at Brookhaven National Laboratory in the USA.) This is where research facilities such as ELENA come in, where substances such as antihydrogen can be artificially produced using pair-forming reactions.
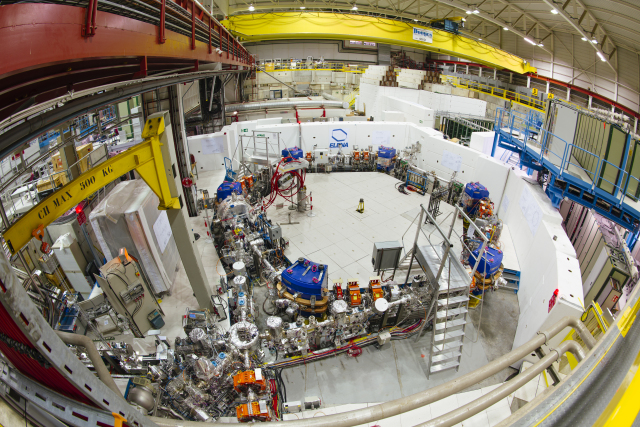
With the help of the ELENA deceleration ring, researchers at CERN hope to uncover the remaining mysteries surrounding antimatter particles.
Source: CERN (Maximilien Brice.)
Generating antiatoms
First of all, this production process requires (negatively charged) antiprotons and (positively charged) positrons. The antiprotons are produced in a special CERN facility by shooting a very energetic proton beam at an iridium target. The resulting antiprotons are then cooled and decelerated in the ELENA deceleration ring, and subsequently confined in magnetic traps. The positrons, on the other hand, come from a radioactive Na-22 atomic nucleus, and they, too, must be cooled and trapped after they emerge. Patience is then required, because in order for the cold antiparticles to combine into an antihydrogen atom, an antiproton and two positrons must be in the right place at exactly the right time (so-called three-particle recombination). Afterwards, the "only" task left is to split off one of the positrons, then the antihydrogen atom is ready!
Of course, the process just described is anything but trivial, and it requires scientific work at the very highest level. In fact, ELENA and the related experiments such as GBAR are another CERN application that fully exploits the realm of the technically feasible. Says Jose Antonio Ferreira Somoza, a research member of the ELENA team: "With this project, we are true pioneers pushing the limits of science!"
Above all, the vacuum valves around ELENA must have extremely high precision and reliability; after all, most of the machine operates under XHV conditions, i.e., in a pressure range between 10-11 and 10-12 mbar. "To achieve such a low vacuum, all components must be free of volatile substances, otherwise the vacuum system would be unacceptably contaminated," Jose Antonio Ferreira Somoza describes the problem. "Accordingly, we have to do extensive bakeout work, and because high heat is used during the baking, we need vacuum components that can withstand the corresponding heat load."
XHV vacuum thanks to VAT all-metal gate valves
Specifically, the ELENA developers chose special all-metal gate valves of the VAT series 48 (DN 63 and DN 100), which have two side ports (DN 40) with CF flanges. "The series-48 all-metal gate valves are designed for reliable shut-off in UHV and XHV applications with high ambient temperatures at the valve of up to 300°C. They can also withstand radiation of up to 108 Gy," says Jürg Öhri, the sales manager responsible at VAT, describing the advantages of this valve series. One of the two ports is used for continuous measurement of the vacuum level around the valve disc. The second port has two functions, depending on the valve’s position. Jose Antonio Ferreira Somoza explains: "If a sector has to be vented for maintenance reasons, we can connect a backing pump via this port to put the sector in question back under vacuum. On the other hand, it also serves us as an injection port for neon gas."
Why Neon? Jürg Öhri knows the technical background here: "In order to reliably achieve the required XHV vacuum range, the inside of the entire deceleration ring had to be coated with NEG (Non-Evaporable Getter). However, when the ring is vented, the problem arises that the NEG coating is very sensitive to oxygen. Therefore, neon gas is introduced first, putting the coating to sleep, so to speak." This technically very demanding process is well worth the effort, as Jose Antonio Ferreira Somoza happily reports: "The NEG coating serving as a UHV pump, in combination with other pumps, enables a tremendous pumping speed!"
Minimal outgassing please!
Another critical point at ELENA is how to keep outgassing processes as slim as possible. As Jose Antonio Ferreira Somoza puts it: “Outgassing is really the absolute crux of this system! The main gas source is hydrogen that diffuses from the metallic parts of the ring, e.g., the valve bellows. That is why almost all the metallic parts that we use for the vacuum chambers must be baked out in vacuum before they can be safely used at ELENA.”
What a multitude of technical and conceptual challenges that the inventors of ELENA had to overcome! But the CERN developers have once again done an excellent job and set another milestone in science with ELENA! ELENA's pre-predecessor LEAR (short for Low Energy Antiproton Ring) had already caused a sensation in 1995, when researchers there first succeeded in producing some antihydrogen atoms. In 2010, CERN researchers managed to produce 38 individual antihydrogen atoms in an improved device called AD (short for Antiproton Decelerator), some of which lasted up to a quarter of an hour. The measurements of the antimatter became more and more precise, and each measurement confirmed Dirac's assumption that matter and antimatter are like each other, except for the charge sign.
ELENA enters the arena
In 2017, the new ELENA deceleration ring was put into operation in order to enable even more precise antimatter studies. The basic idea here is that the slower the antiprotons are, the more precisely their properties can be measured and the more promising are attempts to manipulate them, or move them to form chemical bonds. Compared to the AD layout, the experimental area was not significantly changed, but the much lower beam energies required a radical redesign of the transfer lines. The beam cooling in the new ELENA ring allows for an enormously high deceleration efficiency and an increased phase space density, which increases the number of trapped antiprotons by a factor of 10 to 100. Several experimental stations, e.g., BASE, GBAR, ALPHA, AEGIS, ASACUSA, or PUMA, are coupled to the deceleration ring and thus reliably supplied with low-energy antiprotons of the highest quality by ELENA. Further experimental zones are already being planned…
The great question of asymmetry
And it all began with the Nobel Prize-worthy positron-brainwave of Paul Dirac and the equally ingenious discovery of the positron by Carl David Anderson, which was also honored with the Nobel Prize in Physics in 1936. But (at least) one more brainwave of the same category will be necessary to finally answer a very central question about antimatter: the question why there seems to be more matter than antimatter in our universe despite the obvious symmetry between matter and antimatter. The cause of this imbalance is one of the last great mysteries of elementary particle physics. In other words: Why does matter as we know it persist for a comparatively long time and not get captured by its associated antiparticles and crumble into a burst of energy?
The simplest hypothesis would be, of course, that it is not a matter surplus at all, but that the associated antimatter has been hiding in distant corners of the universe, e.g., as galaxies composed entirely of antimatter. But these days, scientists can exclude this hypothesis on the basis of the modern cosmological models to a large extent. Rather, most researchers today assume that matter and antimatter were created in equal amounts during the Big Bang, and that the apparent excess of matter resulted from an extremely weak symmetry breaking in the early stages of the universe – so weak that for every billion particle-antiparticle pairs, there was just one excess particle. Even this minimal imbalance provides a plausible explanation for the excess of matter we find in the universe. In this sense, we all owe our life to this mini-mini-surplus.
Which symmetry was broken?
However, there is disagreement about the background of this symmetry breaking. It cannot be derived from the standard model. Originally, it was assumed that all physical/chemical processes with antiparticles proceed in the same way as with normal particles, in other words, one assumed an invariance of the physical laws under charge conjugation, or C-invariance for short. But soon experiments with neutrinos showed that the weak interaction is not C-invariant. Curiously, an additional swapping of the spatial directions just cancelled the discovered violation of the C-invariance, so that at least the so-called CP-invariance remained. (The P comes from the term "parity" for space reflections.)
But the peace did not last long, because in 1964 a research team around the American physicist James Christenson observed a violation of the combined CP symmetry in the decay of so-called K-mesons (for which they were awarded the Nobel Prize in Physics in 1980). Soon, with the B mesons, further exotic particles were found in which the CP symmetry is broken. Many scientists thought at that time to be quite close to a solution in the question of the asymmetry between matter and antimatter! But then it turned out that this CP phenomenon is much too weak to be the sole cause for the observable asymmetry in the universe.
We can rely on CPT invariance
At least one symmetry proved beyond all doubt, and that is the CPT invariance. The famous CPT theorem, also called Pauli-Lüders theorem after its discoverers, states that all physical laws are invariant to a simultaneous change of sign of the charge (C), a space reflection (P) and a reversal of the time direction (T). This CPT invariance has so far proved to be irrefutable. In 2012, for example, in the BaBar detector at the SLAC Research Institute in California, where collisions of electrons and positrons are studied in detail, it was shown for the first time that in CP-violating processes such as said K-meson decay, T-invariance is also violated and thus the CP violation just cancels out. And in 2016, researchers at CERN succeeded in studying the 1s-1s transition of antihydrogen in detail and proved an extremely high agreement with the corresponding spectral lines of hydrogen. This can be seen as further evidence for the validity of the CPT theorem.
Today, researchers assume that a future theory of elementary particles will be CPT invariant, and that CP invariance is at least a necessary condition for the apparent asymmetry of matter and antimatter. The scientific experiments around ELENA are expected to successively reveal the remaining pieces of the puzzle, which will then give us a comprehensive answer to the big question about matter-antimatter asymmetry.
New opportunities thanks to ELENA
One promising approach that CERN researchers are pursuing has to do with gravity. Not least because of the comparatively weak effect of the gravitational force, it has not yet been particularly well researched how antimatter behaves in gravitational fields, and whether it might react somewhat differently than matter. With the nearly resting (neutral) antihydrogen atoms built from ELENA’s low-energy antiprotons, and with increasingly better measurement methods, it is now possible to study the effects of gravity in ever greater detail. While researchers assume with a high degree of certainty that antiparticles do not fly upwards under the effect of gravity, even the smallest deviations could make the crucial difference!